Designing and fabricating smart biomaterials with precision-engineered characteristics for cutting-edge medical applications.
How are we designing next-gen smart biomaterials?
Advanced engineered smart biomaterials represent a significant breakthrough in the field of precision medicine. These materials are designed to respond dynamically to their environment, providing tailored therapeutic solutions that enhance patient outcomes. Unlike traditional biomaterials, which often serve passive roles in the body, smart biomaterials can actively participate in healing processes, adapting their properties in response to specific biological signals. This responsiveness makes them particularly valuable in developing targeted therapies that address the unique needs of individual patients. One of the key advantages of smart biomaterials over traditional options is their ability to incorporate functionalities such as shape memory, bioactivity, and biodegradability. In cardiovascular applications, for instance, smart biomaterials are utilized in the design of stents and grafts, where maintaining optimal shape and flexibility is critical for ensuring proper blood flow and reducing the risk of complications. These materials can change shape in response to temperature or pH, allowing for better integration with surrounding tissues and improved clinical outcomes. Additionally, they are being explored in the new generation of osteochondral implants, where their advanced properties can significantly reduce implant failure rates by mimicking the natural biomechanics and chemistry of bone and cartilage.
The chemistry of advanced engineered smart biomaterials is foundational to their performance and application. These materials often incorporate various polymeric structures, such as hydrogels and thermoplastic elastomers, which can be chemically modified to achieve desired mechanical and biological properties. For example, polyethylene glycol (PEG) and polycaprolactone (PCL) are commonly used due to their biocompatibility and tunable degradation rates. Moreover, incorporating bioactive molecules or growth factors into the matrix can enhance cellular interactions, promoting tissue regeneration and repair.
Looking to the future, the potential applications of advanced engineered smart biomaterials are vast and promising. As research and development in this domain continue to evolve, we envision innovations that will revolutionize how we approach precision medicine. Our active involvement in this field aims to push the boundaries of biomaterials technology, leading to new solutions for complex medical challenges. By integrating cutting-edge science with practical applications, we strive to contribute to the advancement of healthcare through the development of smarter, more effective biomaterials.
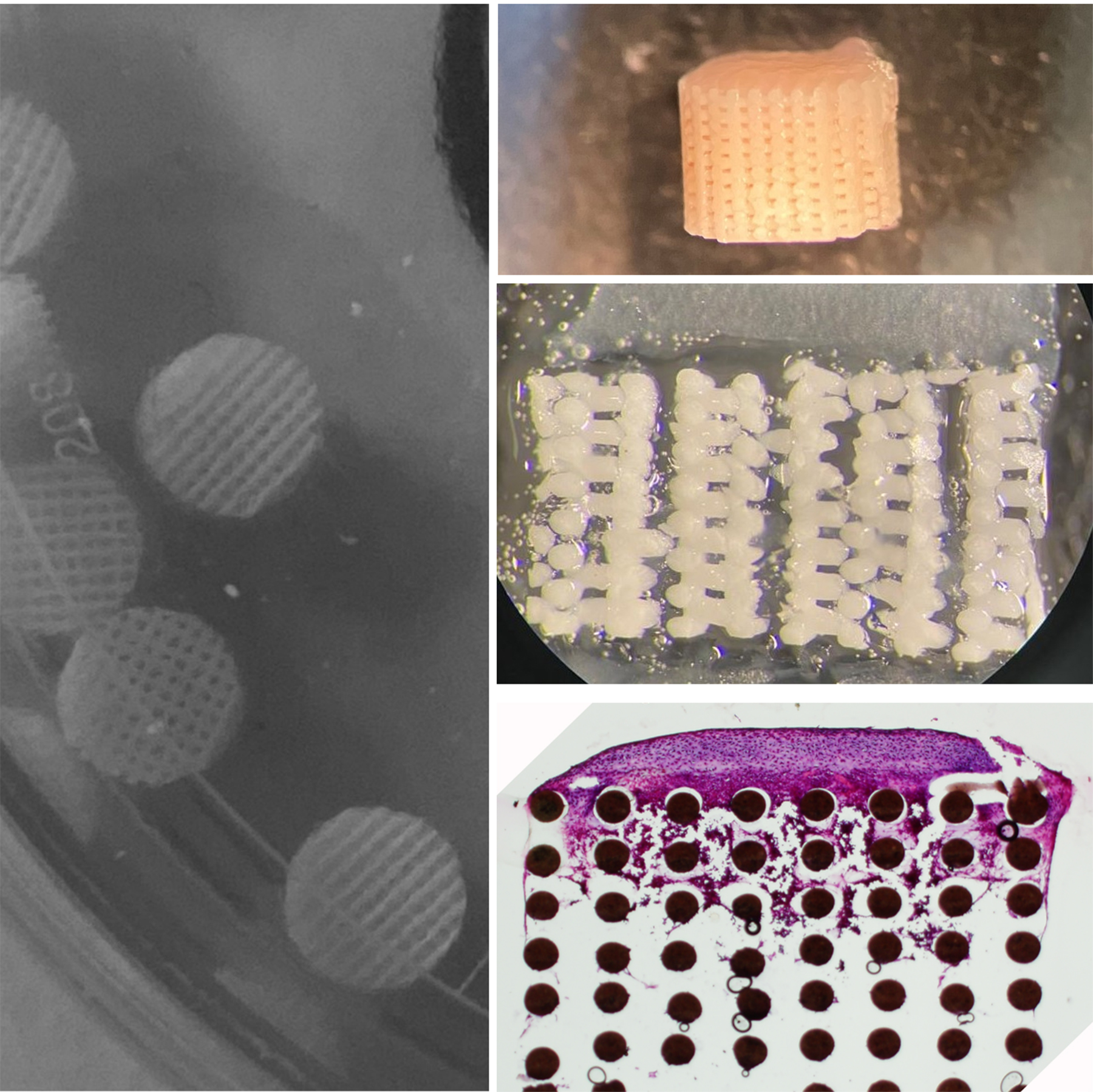
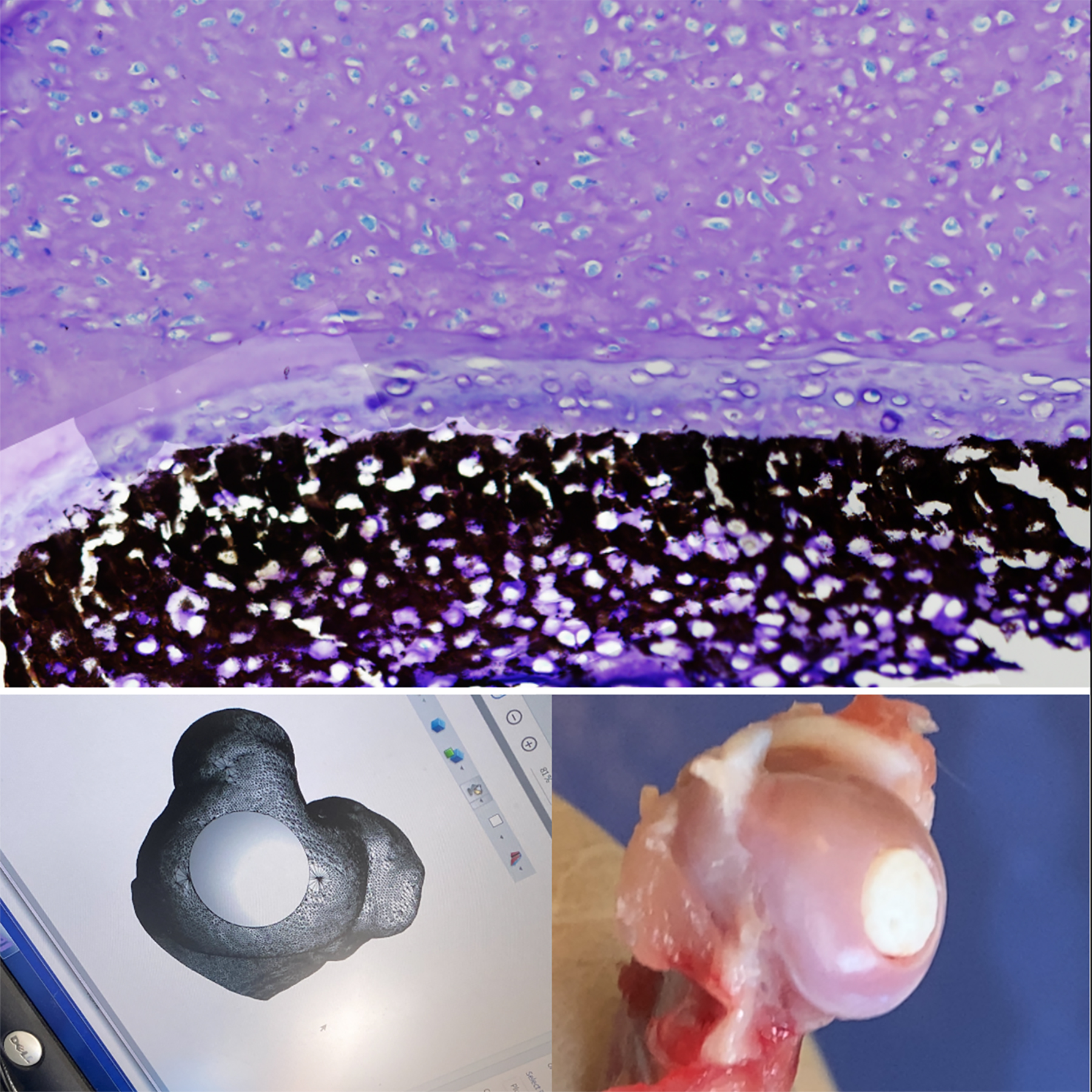
Advancing Smart Biomaterials: Our Collaborative Efforts in Molecular Design and Fabrication Techniques
The development of shape-dependent smart biomaterials represents a significant advancement in the field of biomedical engineering, particularly for applications where mechanical stability and adaptability are crucial. In many implantable devices, such as cardiovascular implants and osteochondral scaffolds, micromotions induced by physiological forces can lead to the formation of microcracks within the material. These cracks not only compromise the structural integrity of the implant but also serve as potential gateways for infections, ultimately resulting in implant failure. Therefore, the ability to design materials that can respond dynamically to mechanical stimuli is essential for improving long-term clinical outcomes.
Smart biomaterials that exhibit shape-dependent properties are engineered to adapt their morphology in response to the biomechanical environment. For instance, these materials can be designed to undergo phase transitions or shape recovery when exposed to specific stimuli such as temperature, pH, or mechanical strain. This adaptive behavior allows them to effectively dissipate stress and accommodate micromotions, reducing the likelihood of crack propagation and subsequent failure. By optimizing the material’s mechanical properties and integrating shape memory effects, these biomaterials can significantly enhance the performance and longevity of cardiovascular implants, ensuring better integration with host tissues.
In the context of osteochondral tissue regeneration, the application of shape-dependent smart biomaterials holds tremendous promise. Osteochondral defects, which involve both cartilage and underlying bone, pose a unique challenge due to the differing mechanical requirements of these tissues. Utilizing advanced biomaterials that can mimic the hierarchical structure of natural osteochondral tissue can facilitate better integration and functional restoration. These materials can be designed to change shape or stiffness in response to physiological loads, thereby promoting proper cellular behavior and tissue regeneration while minimizing the risk of micromotion-induced failure.
Moreover, the chemistry behind these advanced biomaterials is crucial to their performance. Incorporating bioactive agents, such as growth factors or peptides, within the polymeric matrix can enhance the biomaterials’ biological response and support tissue healing. Polymers with tunable mechanical properties, such as poly(lactic-co-glycolic acid) (PLGA) or polyurethane, can be engineered to possess the requisite characteristics for specific applications. By fine-tuning the polymer architecture, degradation rates, and bioactivity, researchers can create smart biomaterials that not only respond to mechanical stimuli but also facilitate the desired biological outcomes.
In conclusion, shape-dependent smart biomaterials offer a transformative approach to addressing the challenges of micromotion-related complications in implantable devices. Their ability to adaptively respond to the mechanical environment positions them as a critical solution for enhancing the performance of cardiovascular implants and facilitating osteochondral tissue regeneration. As research in this domain continues to evolve, these innovative materials will likely play a pivotal role in advancing the future of implantable technologies and regenerative medicine.
Read More
Discover our latest insights and research publications on smart biomaterials